Particle physicists have uncovered a large, hidden void in Khufu’s Pyramid, the largest pyramid in Giza, Egypt – built between 2600 and 2500 BC. The discovery, published in Nature, was made using cosmic-ray based imaging and may help scientists work out how the enigmatic pyramid was actually constructed.
The technology works by tracking particles called muons. They are very similar to electrons – having the same charge and a quantum property called spin – but are 207 times heavier. This difference in mass is quite important as it turns out it determines how these particles interact when hitting matter.
Highly energetic electrons emit electromagnetic radiation, such as X-rays, when they hit solid matter – making them lose energy and get stuck in the target material. Due to the muon’s much higher mass, this emission of electromagnetic radiation is suppressed by a factor of 207 squared compared to electrons. As a result, muons are not stopped so quickly by any material, they are highly penetrative.
Muons are commonly produced in cosmic rays. The Earth’s upper atmosphere is constantly bombarded with charged particles from the sun but also from sources outside of our solar system. It is the latter that provide the more energetic cosmic rays that can produce muons and other particles in a chain of reactions.
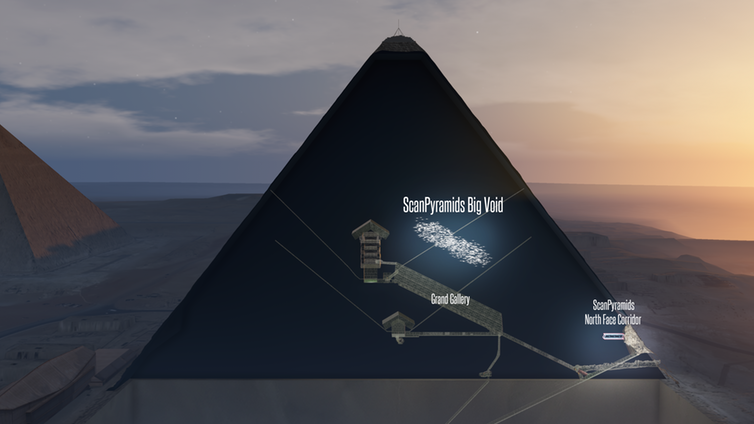
As muons have a relatively long lifetime and are pretty stable, they are the most numerous particles seen from cosmic rays at ground level. And although a lot of energy is lost on the way, muons with very high energies do occur.
Doing science with muons
The particles are fairly easy to detect. They produce a thin trail of “ionisation” along the path they take – which means that they knock electrons off atoms, leaving the atoms charged. This is quite handy, allowing scientists using several detectors to follow the path of the muon back to its origin. Also, if there’s a lot of material in the way of the muon, it can lose all of its energy and stop in the material and decay (split into other particles) before being detected.
These properties make muons great candidates for taking images of objects that otherwise are impenetrable or impossible to observe. Just like bones produce a shadow on a photographic film exposed to X-rays, a heavy and dense object with a high atomic number will produce a shadow or a reduction in the number of muons being able to pass through that object.
The first time muons were used in this way was in 1955, when EP George measured the overburden of rock over a tunnel by comparing the muon flux outside and inside of the said tunnel. The first known attempt to take a deliberate “muogram” happened in 1970 when Luis W Alvarez looked for extended caverns in the second pyramid of Giza, but found none.
Within the last decade or so, muon tomography has experienced a bit of a fresh boost. In 2007, a Japanese collaboration took a muogram of the crater of the volcano Mt Asama to investigate its inside structure.
Muon scans are also being used to investigate the Fukushima reactor remnants. In the UK, the University of Sheffield is proposing to use measurements of the muon flux to monitor carbon storage sites.
Exploring Khufu
The easiest way to use muons to investigate large objects such as a pyramid is to look for differences in the muon flux coming through it. A solid pyramid would leave a shadow or a reduction in the number of muons in that direction. If there is a large hollow void inside the pyramid the muon flux would be increased in the direction of that void. The bigger the difference between “solid” and “hollow” the easier it becomes.
All you need to do is sit somewhere near the ground, look a bit upwards from the horizon towards the pyramid and count the number of muons coming from every direction. As cosmic muons need to be somewhat energetic to pass through a whole pyramid and as our detector “eyes” are relatively small, we need to sit there and count for quite a while, typically several months in order to count enough muons. In the same way as we have two eyes to get a 3D image of the world in our brains, we want two separate detector “eyes” to get a 3D image of the void inside the pyramid.
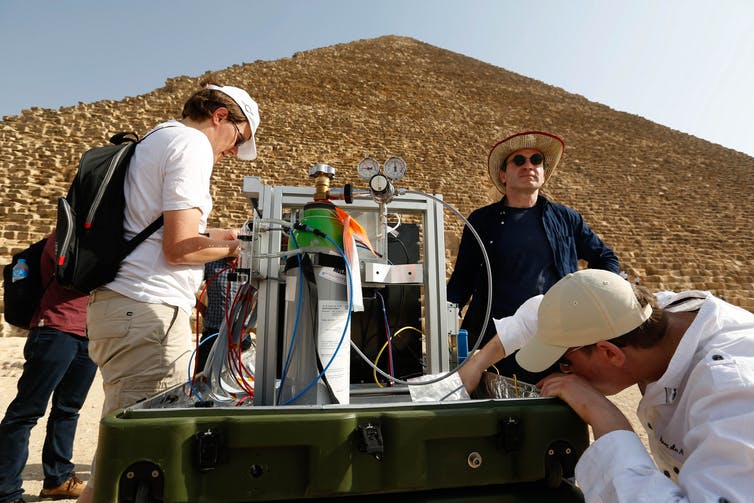
The interesting thing about the approach of this team is that they have chosen three different detector technologies to investigate the pyramid. The first one is a bit old fashioned but offers a supreme resolution of the resulting image: photographic plates which get blackened by the ionisation. These were left for months inside of one of the known chambers in the pyramid and analysed in Japan after data taking was finished.
For the second method plastic “scintillators” that produce a light flash when a charged particle passes through them were employed. These kinds of detectors are used in several modern neutrino experiments.
And finally chambers filled with gas, where the ionisation caused by the charged particles can be monitored, were used to look directly along the direction of the newly discovered cavern.
The electronic signal of those detectors was directly phoned back to Paris via a 3G data link. Of course a pyramid with three known caverns and a large hollow gallery inside is a bit of a complex object to take a muogram of (it only shows light and dark). So often these pictures need to be compared to a computer simulation of the cosmic muons and the known pyramid, with warts and all. In this case, a careful analysis of the pictures of the three detectors and the computer simulation yielded the discovery of a 30 metre long void, up to now unknown, inside of the Great Pyramid of Giza. What a great success for a new toolkit.
The technique can now help us study the detailed shape of this void. While we don’t know anything about the role of the structure, research projects involving scientists from other backgrounds could build on this study to help us discover more about its function.
It’s great to see how cutting-edge particle physics can help us shed light on the most ancient human culture. Perhaps we are witnessing the beginning of a revolution in science – making it truly interdisciplinary.
Harald Fox, Senior Lecturer of Particle Physics, Lancaster University.
This article first appeared on The Conversation.