Lenses revealed the world of the microscopic by letting us see things that were too small for our eyes, giving people like me the chance to become pregnant. That’s just one example, of course; the microscope meant we could study bacteria and viruses, and thereby save lives. At the other end of the scale, lenses in telescopes expanded our view by bringing into focus things that were too far away for our eyes. From believing that the Earth is flat to the realisation that it is but an insignificant dot, one of billions, spread out over inconceivable distances, humans have come a long way in understanding our place in the universe.
Leeuwenhoek left an enduring legacy of looking at little living organisms through a lens. When the Covid-19 pandemic hit, scientists swiftly mapped out the structure of the virus and then raced to create vaccines, so eagerly awaited, and these simply couldn’t have been created without lenses. To battle cancer, another devastating disease that touches so many of us, requires looking as closely as we can at these cells that can carry on replicating forever. But not only are we looking at cancer cells in a highly magnified static view, we are also tracking – in real time – what’s happening inside them.
We can do this by shining a special type of light on the cells: the laser. A laser (the name is an acronym for Light Amplification by Stimulated Emission of Radiation) is an artificially created beam of very pure light. It is extremely powerful – it can cut through metal and pierce diamonds. Laser beams are quite different from the light that comes out of a lightbulb or a torch. These more familiar light sources give off white light – a mixture of light waves of all different colours or wavelengths – but lasers are made up of a single wavelength or a very narrow range of wavelengths. The light from a torch spreads out into a cone and dissipates, but lasers have a tight and narrow beam that’s near parallel, so it travels consistently over much longer distances. The waves of light coming from the torch are mixed up (like people walking around randomly on a street), but the laser waves are synchronised or coherent (like soldiers marching in step).
Another special thing lasers can do is form extremely short pulses in contrast to the continuous waves of light emanating from a torch. The lens isn’t necessarily needed to generate a laser emission. But it – along with its triangular cousin, the prism, and the mirror – is essential for transporting and tweaking the emissions, making sure that the beam coming out of the machine has the right width, intensity, and level of synchronisation for your purpose, turning laser emissions into practical tools. Some of the most advanced and powerful lasers in the world even rely on something that’s a cross between a lens and a mirror to generate the emissions in the first place. The titanium:sapphire laser is one that produces some of the shortest and most powerful laser pulses. To generate these pulses, a green laser is shone onto a titanium:sapphire crystal to excite its atoms. These excited atoms emit pulses of light that are of a longer wavelength (red) and more energetic.
A pair of these mirror/lens devices are arranged in such a way that the green light from the laser passes through them and is focused down to create a high intensity point at the crystal, behaving like a lens. The red light that is emitted from the crystal, however, gets reflected back and forth between the mirrors until they form a strong beam, and is then allowed to pass through into the main instrument. Professor Stanley Botchway uses one of these lasers, which can produce pulses as short as six femtoseconds (or six quadrillionths of a second), as a form of microscope for his research. Stan is investigating how the DNA inside our cells gets damaged when exposed to different types of radiation.
At the moment, broadly speaking, radiotherapy to treat cancer fires high-energy X-rays at tumours to kill the cancerous cells – but the problem is that healthy cells around the tumour are also damaged, causing difficult side effects. One of his projects is testing how to use lasers alongside medication, a process called photodynamic therapy. The medicinal molecules are administered to the patient in an unactivated form, and they seek out cancer cells because they have been designed to prefer environments with less oxygen (tumours grow so fast that the blood vessels don’t have time to catch up, so there’s less oxygen). The drug targets the skeleton of the cancer cells that holds the cells together. Then, the titanium:sapphire laser is carefully pointed at the tumour. When hit by the femtosecond pulses, the drug becomes activated and kills the cancerous cells.
Since the surrounding healthy cells will not have attracted much of the drug, and also because the laser can be so carefully aimed at the tumour, the healthy cells are minimally affected. The red light from these lasers can penetrate deeper into tissue than X-rays, so they are far more effective in treating hard-to-reach tumours while reducing the side effects. It’s worth noting that the cells used in this form of research into cancer, as well as countless other diseases, are called HeLa cells. These are cancer cells that were taken for research without consent from a Black American woman, Henrietta Lacks, who died at the age of 31 in 1951. The cells taken from her have been alive and replicated ever since, and are one of the most important cell-lines in medical research.
Johns Hopkins issued an apology over 50 years later, but her family have received minimal compensation. Scientists are also using these lasers with incredibly short pulses to watch biological processes that happen inside cells. There are interactions or changes that occur in our cells because of disease – and also in healthy cells – so quickly that it’s impossible to see with normal microscopes. By sending these pulses that are a tiny fraction of a second to the cell and taking hundreds of thousands of images every second, we get to watch the building blocks of life in action.
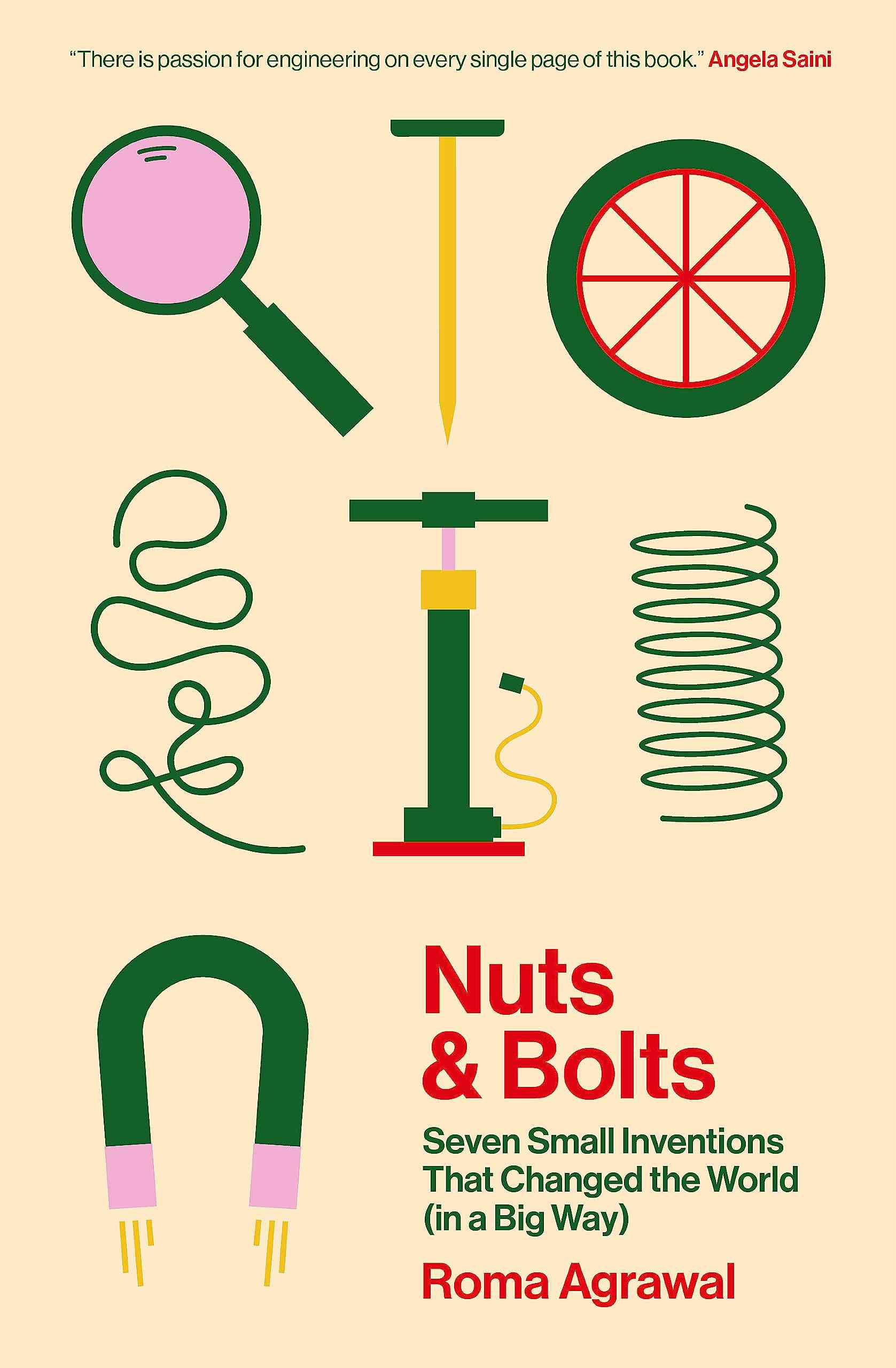
Excerpted with permission from Nuts and Bolts: Seven Small Inventions That Changed the World (in a Big Way), Roma Agarwal, Hachette India.