A vaccine for a new disease usually takes more than ten years to be ready to be given to people. In the case of Ebola, which was fast- tracked, it took five years for a candidate vaccine to go into clinical trials. Vaccine development has moved exceptionally quickly since January when the causative agent of COVID-19 was identified. The first vaccines for COVID-19 were created and went through clinical trials in under a year. This was a record and was recognised by Science as the “2020 Breakthrough of the Year”.
How was this even possible without cutting corners?
Many of the traditional steps of vaccine development were contracted. Initially, many assumptions were made in the development of a SARS-CoV-2 vaccine based on experience with other viruses. Vaccine makers learned from the experience with Ebola. In many ways, the world was fortunate that we were dealing with a coronavirus, because there were vaccine-development plans under way for MERS, another coronavirus.
Additionally, many new technologies and platforms were used that increased the speed of vaccine development. This is in contrast with the earliest generation of vaccines for other diseases which used a more passive approach. I’ll discuss these technologies later in this chapter.
By the end of 2020, there were multiple vaccines that had gone through the gauntlet of clinical trials and had received provisional approvals for use in many parts of the world. While these vaccines were still in clinical trials, manufacturers had already initiated the process of producing them in the hope that they would work. Two mainstream frontrunner vaccines showed similar efficacy (around 95 per cent) in preventing disease after two doses – a prime and a booster shot.12 Other vaccines also demonstrated that they worked with slightly lower efficacy.
Fundamentally, COVID-19 vaccines are trying to do the same thing. They hinge on the idea of preventing the spike protein from binding to the ACE2 receptor. This is the key step in infection, and while antibodies can form to other viral proteins, the neutralising antibodies we want are geared towards this interaction. The virus isn’t completely defenceless against antibodies either: it is coated with sugars and tries to keep the crucial part of the spike covered up so that antibodies can’t access it.
Traditionally, a vaccine was created from virus that was isolated and weakened or inactivated.
The whole virus (or a part of it) was inserted into the body (which made neutralising antibodies). This is the basis for most vaccines in use.
Within months of the identification of SARS-CoV-2, there were vaccine candidates that used weakened virus, killed (inactivated) virus or pieces of the virus to try to generate an immune response. This is remarkable not only for the speed at which the vaccine candidates were created, but also because they represent so many different strategies.
Without going into the debate of whether viruses are living in the first place, colloquially vaccines with inactivated viruses are “killed” by chemical treatment or heat. What this means is that after they are injected into the body, they can fire up the immune system but can’t replicate inside cells. Whole-inactivated virus vaccines require an additional substance to boost the immune response. This is called an adjuvant.
On the other hand, instead of inactivating the virus, a vaccine can also consist of weakened virus to the extent that it causes only a mild infection, while losing all its disease-causing properties. Traditionally, weakened viruses were generated by passing through cell cultures repeatedly.
The safety of inactivated and live virus vaccines is tested extensively since they use actual viruses.
Large amounts of virus are required for inactivated viruses. For weakened live viruses, we must make sure that they do not revert to their disease-causing ancestral strains. Again, because a live virus is being injected, extensive testing is necessary to ensure that these are safe for those with compromised immune systems.
An example of an inactivated vaccine is Covaxin which was created in India by the National Institute of Virology of the Indian Council of Medical Research, and Bharat Biotech. Thousands of participants were being enrolled for a Phase III clinical trial in late November 2020.
Other vaccines can be made from protein parts of viruses or virus-like particles, all of which are deficient in some key component present in an infectious virus. Some of these also require adjuvants to fire up the immune system.
A few vaccines consist of virus proteins packed into nanoparticles and injected into the body. These virus proteins are recognised as alien by the immune system which starts to mount an appropriate response. By the end of 2020, Novavax had enrolled patients in Phase III clinical trials for their version of this kind of vaccine.
Another approach relies on creating a “virus-like-particle” that mimics SARS-CoV-2 but doesn’t have any genetic material inside. These are expected to cause the body to mount an immune response, but since they have no genetic material, they aren’t infectious. However, the vaccines approved by the end of 2020 and most of the other candidate vaccines are using platforms that do not require actual virus or parts, but instead use genetic information to get human cells to create the virus parts much like the virus would.
These are rapid and safe systems.
DNA or RNA is injected into the body where it serves as a blueprint for the cellular machinery to make parts of a virus that are stuck on antigen-presenting cells. The immune system recognises these cell-created virus parts as being “alien” and makes antibodies against them. Prior to this pandemic there had been limited experience with working with these systems, although some of these platforms had reasonable success with cancer and other molecular diseases.
Some companies are using the route of a DNA vaccine with genetic material in the shape of a garland. Getting DNA inside cells has been somewhat of a problem. So, a technique called electroporation, which employs electricity to briefly create holes in the membrane of cells, is being used. Once inside the cell, the DNA is transcribed into RNA which is translated into the coronavirus spike protein that can hopefully cause people to generate a strong immune response to it.
But by the end of 2020, the vaccines that had generated the most excitement used RNA.
An RNA vaccine skips the first few steps of a DNA vaccine entirely and induces potent immunity. Once inside the cell, it is translated directly into the spike protein which is used by the host cell to build up immune responses.
By the end of November, two leading candidate vaccines created by Pfizer and BioNTech and by Moderna that used RNA technology had been shown to be effective after two doses. In December, these candidates were the first two mainstream vaccines approved in the world. The broad use of mRNA vaccines may signal a paradigm shift in how we try to prevent the spread of infectious diseases.
Why are the first approved RNA vaccines only available now? After all, they could’ve helped us to combat other infectious diseases in the past. Three recent technical advances make these vaccines possible. First, RNA is unstable and difficult to get inside cells. But by encasing it in molecules known as lipid nanoparticles, delivery and stability have been improved. Second, “foreign” RNA can trigger an immune response (instead of the protein that it helps the body make). But if the RNA is chemically made with synthetic nucleosides, then the immune system doesn’t react against it. Third, RNA is “read” by the host cells to make viral proteins. But before it was modified and stable, it was not read well. The proverbial stars aligned shortly before the COVID-19 pandemic.
RNA vaccines are not without downsides, however. RNA is less stable than many other biological molecules.
Enzymes that can degrade RNA are ubiquitous. Even with chemical changes that improve stability, the Pfizer vaccine has to be kept at -70°C. Moderna’s vaccine is more stable and can be kept in a regular freezer at -20°C for six months.
The ability to produce and distribute hundreds of millions of doses of RNA vaccines during an active pandemic remains challenging. But the good news is that by the end of 2020, clinical trials and preliminary observations following weeks of broader roll- out indicated that both approved RNA vaccines were generally well tolerated. Some adverse effects such as pain at the site of injection, tiredness, headaches or mild fever had been reported, but there were no widespread safety concerns.20
The last class of vaccines I want to discuss contain a DNA blueprint inserted into the shell of a harmless virus (usually an adenovirus vector). This is quite ingenious in that it uses a defective virus to deliver a message that will generate antibodies to SARS-CoV-2. These defective viruses generate immune responses but are either too weak to cause disease or are missing the necessary components to replicate altogether.
In 2020, AstraZeneca, in conjunction with Oxford University, and Johnson & Johnson were two companies that had enrolled thousands of participants in trials of vaccines that use adenovirus vectors. By late 2020, the AstraZeneca candidate vaccine had shown promising, initial results and was poised for approval for use in 2021.
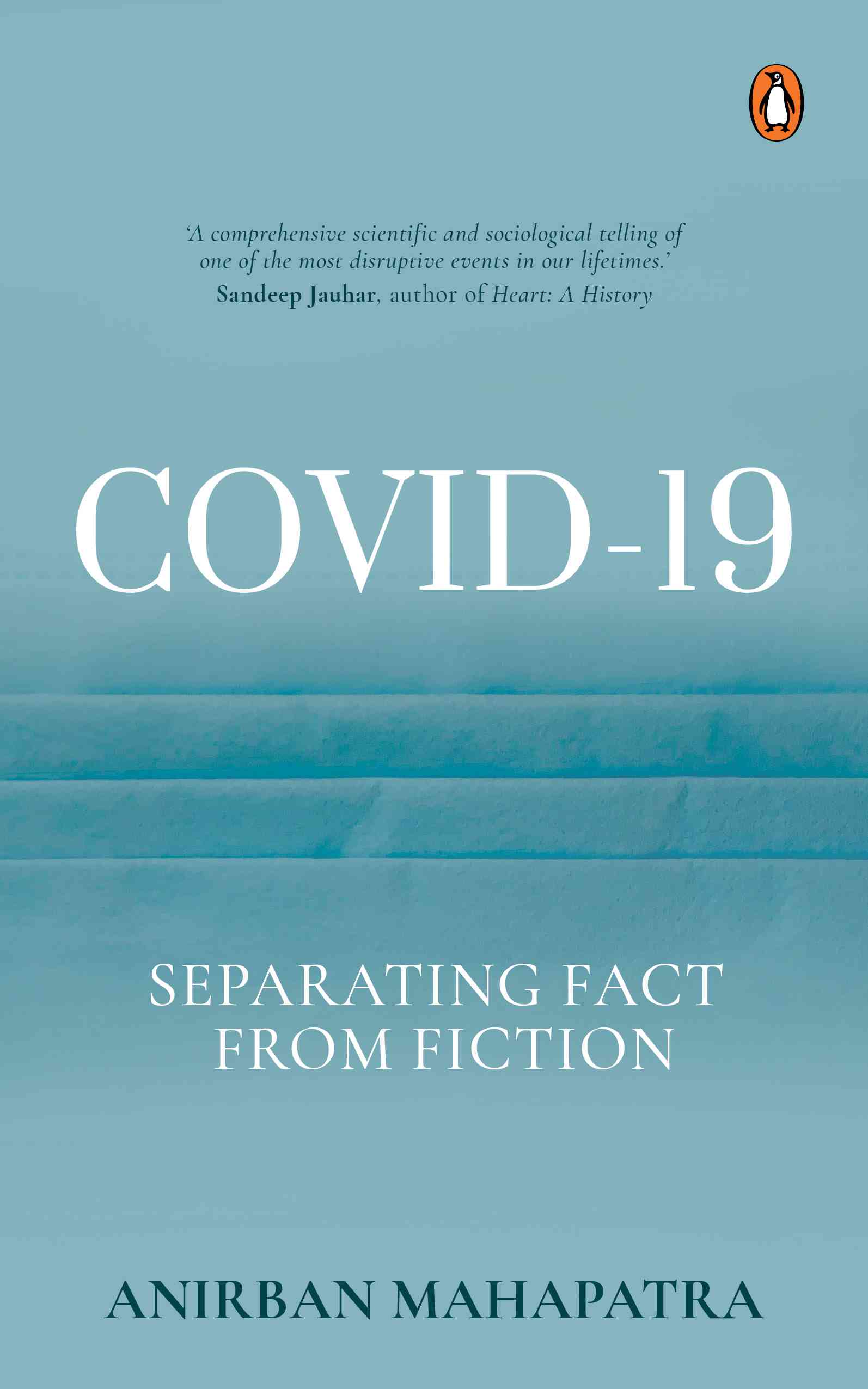
Excerpted with permission from Covid-19: Separating fact From Fiction, Anirban Mahapatra, Penguin Books.