Heart disease has become the leading cause of death in India in recent years, now responsible for a quarter of all mortality in the country. Ischemic heart disease – reduced blood supply due to thickening of arteries, often caused by high cholesterol levels – and stroke are responsible for more than 80% of all cardiovascular deaths. This, despite there having been several therapeutic advances in treating cardiovascular diseases over the decades.
One approach that scientists have been taking in order to understand ischemic heart disease better is to examine how exactly the structure and elasticity of blood vessels impact blood flow and function. Blood flow in the body is generally laminar, which is a smooth orderly movement of blood in one direction. Under certain conditions, like while ascending into the aorta, blood flow becomes turbulent or chaotic.
More than a decade ago Viswanathan Kumaran decided to take up theoretical research to examine the effect of fluid flows in soft walled tubes, not unlike blood vessels in the human body. Kumaran is a professor at the department of chemical engineering at the Indian Institute of Science in Bangalore. Kumaran and his colleagues made predictions of how fluid flows change in soft-walled tubes and then carried out experiments to verify these predictions. The work resulted in a model that might be applied to gauge the severity of disease in cardiac patients, the progress of the disease and how to treat them.
He has also applied the same findings in fluid dynamics to help develop lab-on-a-chip devices that can allow diagnostic services to be taken to a patient, instead of having a patient come to a lab. The lab-on-a-chip could vastly improve diagnosis of disease in rural India where both diagnostic services and treatment can be difficult to access.
Kumaran, who was awarded the Infosys Prize in 2016 for his work, spoke to Scroll.in. Here is an excerpt from that conversation.
When you first took up this research more than 10 years ago, what aspect of fluid dynamics were you looking at?
Normally, fluids flow in two different ways. One is what is called a laminar flow, where they flow in a nice smooth sort of way and the other is turbulent flow, which is chaotic and intermittent. The transition between these can in some cases be predicted theoretically. This is particularly important because most flows, in industry and so on, are turbulent. This has consequences in the physiological system as well where the shearing of the arterial wall which causes many cardiovascular conditions depends critically on the nature of the flow of blood in arteries. That was our original motivation of getting into this area.
[Shear stress is a mechanical force that blood applies along the inner walls, or endothelium, of blood vessels as it flows. The wall, on the other hand, applies an opposing friction-like force on the blood. Low shear stress that is caused by laminar flow allows endothelial cells to survive longer and secrete substances that promote dilation of blood vessels and prevent blood clotting. High shear stress or changing shear stress direction as during turbulent flow allows endothelial cell proliferation, shape change, and secretion of substances that constrict blood vessels, promote blood clotting and cause blood platelets to aggregate.]
How did you go about trying to estimate how flows changed from laminar to turbulent in different systems?
Turbulent flows cause more shear and there is more stress on the wall. But turbulent flows are also more efficient in mixing things. For example, in mixing oxygen into the blood flow or in microfluidic applications if you want to mix two fluids for sample preparation, turbulent flows mix things 1,00,000 times to a million times faster than laminar flows. Basically, what we were predicting was at what speed flow is going to change from laminar to turbulent.
Previously all of the work that was done presuming that the walls of a blood vessel are deformed but rigid. Like good theoreticians, we also assumed that the channel or the pipe is straight, the walls are not deformable and so on. That worked in certain situations where we were able to make quantitative predictions. But when you pump fluids through a soft channel it is going to bulge at the entrance and we did not take that into account and we found that we were off by a factor of 10. The work that we are doing is based on walls that are deformed and flexible taking into account the coupling between wall dynamics and flow dynamics. Then, we found that our predictions were accurate.
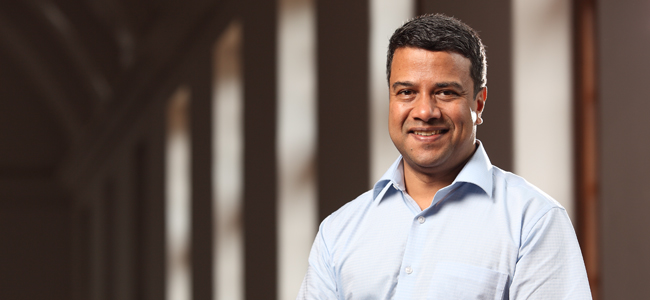
How is this applied to the physiological system?
Cardiovascular flow is influenced by the shape of an aorta or some such complex geometry within the body, then by wall configuration like the presence of hardening and the presence of cholesterol. Based on these there is going to be a change in the mechanical properties of the wall. What we are trying to see is that whether such changes to mechanical properties can cause transition of laminar blood flow to turbulent flows with significantly high shearing. The end goal is that if we get an Magnetic Resonance Imaging or MRI scan or Computerised Axial Tomography or CAT scan of a patient, we should be able simulate his blood flow. Then, we can predict, for doctors’ information, the patient’s blood flow regime and if the doctor performs certain interventions (medicines or surgical procedure) how that would change the patient’s outcomes.
We still need to get actual information from patients to see if we can get the shape of the wall as it is deforming as the blood pumps through. Once we get these velocity profiles from actual patients then we can make quantitative predictions. Right now, the challenge is to get flow profiles and properties of the blood vessel walls with sufficient accuracy that we can go ahead and make a prediction of how turbulent flows will take place and how they will affect the patient.
So right now you have a way of simulating blood flows in different kinds of blood vessel walls and once you have patient profiles, you can put in their physiological information and then make predictions on how disease might progress.
Yes. The technology has to lead to making personalised predictions of cardiovascular health.
What comes next? Are you working with medical researchers? Will there be a clinical trial?
We are trying to establish collaborations with medical researchers. First, we have to see how we can import all the information they have from MRI scans and so on into the fluid dynamics solver. Once we have that, we will have to see how to do the simulations.
This will have to be large-scale research to get enough patient profiles to test the accuracy of the fluid dynamic model. It also depends on advances in computing power to make these simulations possible.
What is the lab-on-a-chip?
Lab-on-a-chip is a device like a glucose meter where, if you can take a drop of blood and put it on this chip that might be the size of a credit card, then you can get a whole range from results like blood cell count and that kind of thing. While glucose meters work on just on capillary action (a glucose meter has a sensor that that measures the level of glucose in the blood over time and translates that into an electrical signal), getting a blood cell count involves three or four steps of sample preparation.
How is the lab-on-a-chip application connected to this work in fluid dynamics?
For a lab-on-a-chip, laboratory operations are done on a very small scale. There are already devices in the market like glucose meters but they do not involve sample preparation – putting two fluids together, mixing them, waiting for some time and putting in another fluid and so on. Those operations are traditionally done in diagnostic labs where you can put two fluids in a test tube and shake it up. But when you have a channel on a chip that is the size of human hair, flow is almost always laminar. So mixing takes a long time – of the order of 10 minutes or so. What we are trying to do is to introduce turbulence and reduce the mixing times to about tens of milliseconds, so that sample preparation can be done in a way that can be reproducible.
I provide technical input for a the work at a company called MicroXLabs, which was incubated at the Indian Institute of Science, that has already made working devices. They are now at the stage of testing how it can be manufactured. Even if we can produce something in the lab, to actually have it working on the ground we have to produce it in an efficient way.